Improving FPGA Debugging with Assertions
Here’s one reason why FPGA design starts dwarf ASIC design starts: choosing flexible, inexpensive and readily available FPGAs is one fairly obvious way to reduce risk when designing complex SoCs for everything from mobile devices and smartphones to automobile electronics.
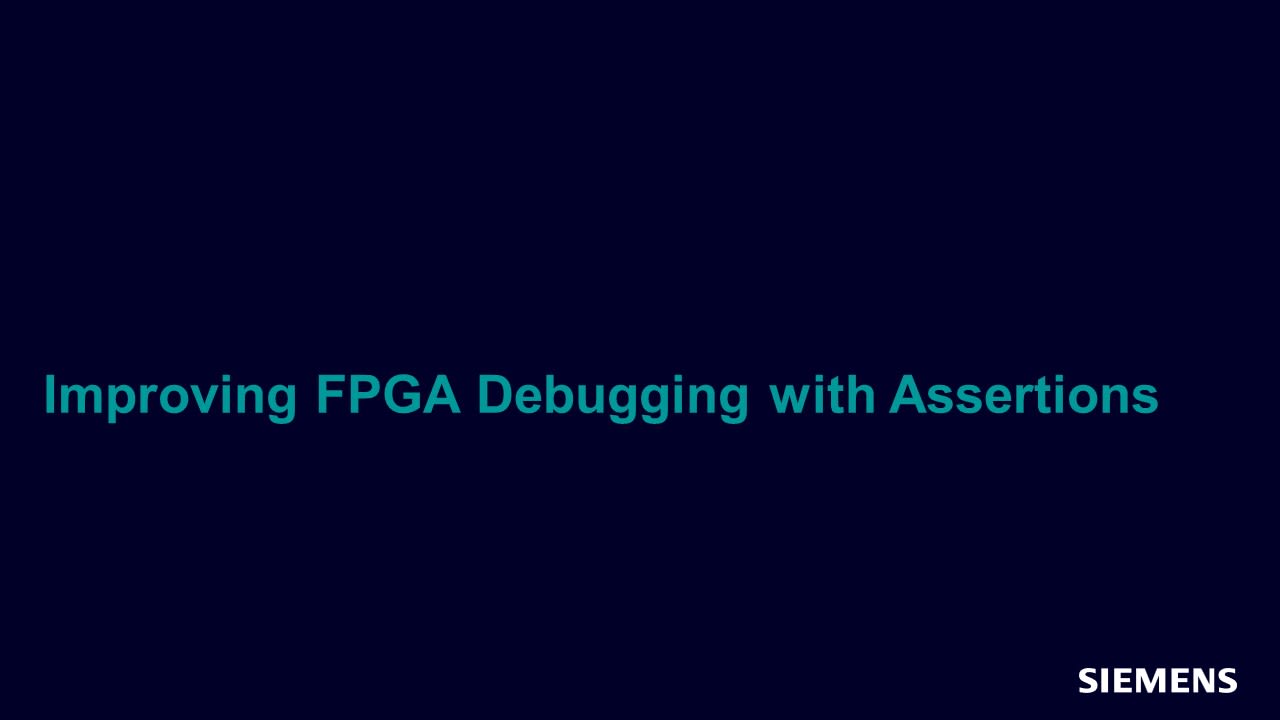
Full-access members only
Register your account to view Improving FPGA Debugging with Assertions
Full-access members gain access to our free tools and training, including our full library of articles, recorded sessions, seminars, papers, learning tracks, in-depth verification cookbooks, and more.